1. Introduction: Batteries Play an Important Role in Decarbonization
In the face of climate change, the shift toward decarbonizing our energy usage and utilizing renewable energy resources has become essential. As with the other technologies listed on the Qurator Decarbonization Marketplace, batteries play a key role in this process because of their ability to efficiently store and deliver energy. But not all batteries are the same — the chemistry occurring inside a battery greatly affects its characteristics and use cases. This article reviews the key battery chemistries relevant today, presenting an overview of how each works and its advantages and limitations.
Battery Chemistry in a Nutshell
All batteries rely on the basic principle of converting stored chemical energy into useful electrical energy through electrochemical reactions. This is accomplished using one or more electrochemical cells, which consist of two electrodes — a negative electrode (anode) and a positive electrode (cathode) — separated by an electrolyte.
The electrochemical reactions that occur during battery operation produce electrons at the anode and consume them at the cathode. This generates a flow of electrons from the anode to the cathode through the external circuit, which we harness to power devices ranging from smartphones to electric vehicles.
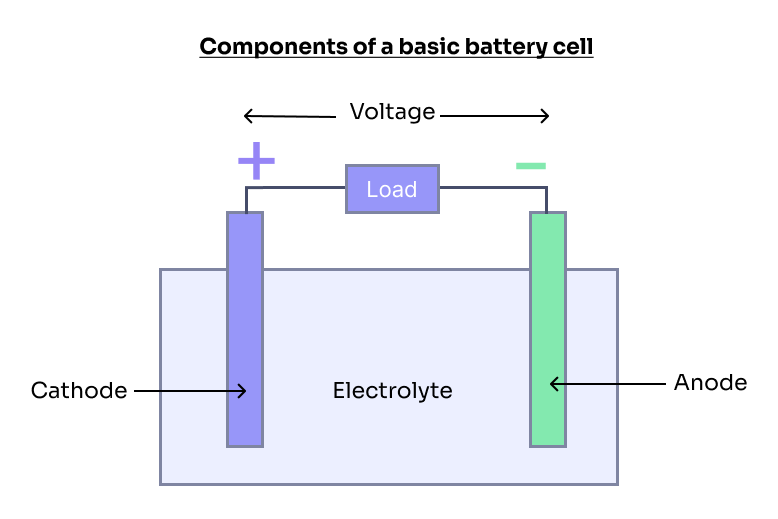
In a nutshell, the materials selected for the anode, cathode, and electrolyte together comprise what is commonly referred to as "battery chemistry." This combination defines the battery's performance characteristics, such as energy density, power density, lifespan, safety, and environmental impact.
2. Traditional Battery Chemistries: Lead–Acid and Nickel-Based
Starting from the roots, we first explore three traditional battery chemistries. Lead–acid batteries are one of the oldest types of practical batteries but remain relevant for automotive starters and backup power systems even today, while nickel–cadmium (NiCd) and nickel–metal hydride (NiMH) batteries have each played their part in the path to developing robust and reliable energy solutions.
Lead–Acid: Bridging the Old With the New
The lead–acid battery, invented by French physicist Gaston Planté in 1859, is one of the oldest rechargeable battery types. Its reliability, low cost, and ability to deliver high surge currents have ensured its continued use in various applications since its inception.
Lead–acid batteries utilize electrochemical reactions between lead-based electrodes and sulfuric acid to convert chemical energy into electrical energy. These reactions facilitate the flow of electrons from the anode to the cathode through the external circuit, enabling functions like starting cars.
Other than starting cars, lead-acid batteries are commonly used as dependable, cost-effective backup power for essential services such as hospitals. They also power industrial machinery and marine vehicles, although newer technologies are challenging their role in renewable energy storage systems.
Despite their widespread use, lead–acid batteries have environmental drawbacks due to the toxicity of lead and the corrosive nature of sulfuric acid. Recycling programs and regulations are in place to mitigate these environmental impacts.
>> Check out the vendors in lead-acid battery manufacturing on Qurator.com here
Nickel–Cadmium: The Robust Warrior
One of the earliest rechargeable battery types to see widespread consumer use, nickel–cadmium batteries use a cathode made of nickel oxide hydroxide, an anode made of metallic cadmium, and an alkaline electrolyte, typically potassium hydroxide. During discharge, the cadmium anode is oxidized to cadmium hydroxide with the release of electrons, while the nickel oxide hydroxide cathode is reduced to nickel hydroxide using these electrons.
Known for their robust performance and high durability, nickel–cadmium batteries have a moderate energy density but can suffer from the memory effect, where the battery "remembers" its discharge history, leading to a decrease in its maximum energy capacity if it is not fully discharged before being recharged. Moreover, cadmium is a toxic heavy metal and, coupled with ongoing technological advances, this has led to these batteries being replaced by other battery types in many applications.
>> Check out the vendors in nickel-cadmium battery manufacturing on Qurator.com here
Nickel–Metal Hydride: The Transition to Higher Energy
Nickel–metal hydride batteries have a similar chemistry to nickel–cadmium batteries but use a hydrogen-absorbing alloy as the anode rather than toxic cadmium. Because of environmental considerations and their higher energy density, nickel–metal hydride batteries have now largely supplanted nickel–cadmium batteries in most applications, such as consumer electronics.
These batteries were also employed in most early-generation electric and hybrid electric vehicles, although they have now been almost entirely superseded by lithium-ion batteries for all-electric vehicles and plug-in hybrids.
>> Check out the vendors in nickel-metal hydride battery manufacturing on Qurator.com here
3. Current Champion in the Race: Lithium-Ion Batteries
In our quest for greener renewable energy storage solutions, lithium-ion (Li-ion) batteries have emerged as the current champions. Because of their high energy density, long lifespan, lack of memory effect, and slow loss of charge when not in use, lithium-ion batteries have become the dominant technology for portable electronics, electric vehicles, and numerous other applications.
Lithium Ion: Powering the Present
It is difficult to overstate the impact that lithium-ion batteries have had on modern life. Their discovery — which led to the inventors being awarded the 2019 Nobel Prize in Chemistry — has enabled the development of mobile phones, laptops, and other portable electronics that are now integral to daily activities, transforming the way we communicate, work, and access information.
Furthermore, by powering electric vehicles and providing opportunities to store renewable energy, lithium-ion batteries are at the forefront of the shift toward cleaner transportation and power generation, significantly contributing to efforts in reducing global carbon emissions and combating climate change.
A bewildering array of lithium-ion battery chemistries are now available, each with its own advantages and disadvantages. The most common chemistries include lithium cobalt oxide (LCO), lithium manganese oxide (LMO), lithium nickel manganese cobalt oxide (NMC), lithium iron phosphate (LFP) (read also: How long do lithium iron phosphate batteries last?), and lithium nickel cobalt aluminum oxide (NCA). These vary in the choice of cathode material, which is a key part of the battery responsible for storing and releasing lithium ions during charging and discharging. The anode is typically based on graphite.
During discharge, lithium ions move from the anode to the cathode through the electrolyte, while the released electrons move in the same direction through the external circuit. This is made possible by the ability of both electrodes to permit the movement of lithium ions into and out of their structures through a process called intercalation. During charging, the lithium ions move in the opposite direction back toward the anode.

>> See all battery manufacturing vendors on Qurator.com here
Lithium Polymer: The Flexible Alternative
Lithium polymer (LiPo) batteries have evolved from lithium-ion batteries, with the primary difference being the use of a polymer electrolyte rather than a liquid electrolyte. These provide a higher specific energy than other types of lithium-ion batteries, making them virtually ubiquitous in applications where weight is critical, such as mobile devices and drones. They can also be fabricated in a variety of shapes and sizes with a lower chance of electrolyte leakage. However, they tend to be more expensive and have a shorter lifespan.
>> See all battery manufacturing vendors on Qurator.com here
4. Future Battery Chemistries: Solid-State and Beyond
Building a sustainable renewable energy environment requires imagining the future. As the demand for safer, more efficient, and higher-capacity energy storage solutions grows, these emerging technologies promise to redefine the capabilities of batteries for a diverse array of applications, from electric vehicles to large-scale energy storage systems.
Solid-State Batteries: A Glimpse Into the Future
Solid-state batteries represent a major leap forward from traditional lithium-ion batteries, primarily due to their use of a solid electrolyte instead of a liquid one. This fundamental change in design offers several advantages, including higher energy densities, improved safety, and longer lifespans. By omitting the volatile and flammable liquid electrolytes often used in conventional batteries, solid-state batteries significantly reduce the risk of leaks and fires, addressing one of the major safety concerns associated with current battery chemistries.
Moreover, solid-state batteries can potentially achieve higher energy densities by utilizing lithium metal anodes instead of the graphite commonly found in lithium-ion batteries. This could lead to batteries that not only charge faster but also hold more energy, opening up new possibilities for electric vehicles by extending their range and reducing charging times. Despite these promising features, challenges such as high manufacturing costs, material compatibility, and the need for advanced manufacturing techniques remain hurdles on the path to widespread adoption.
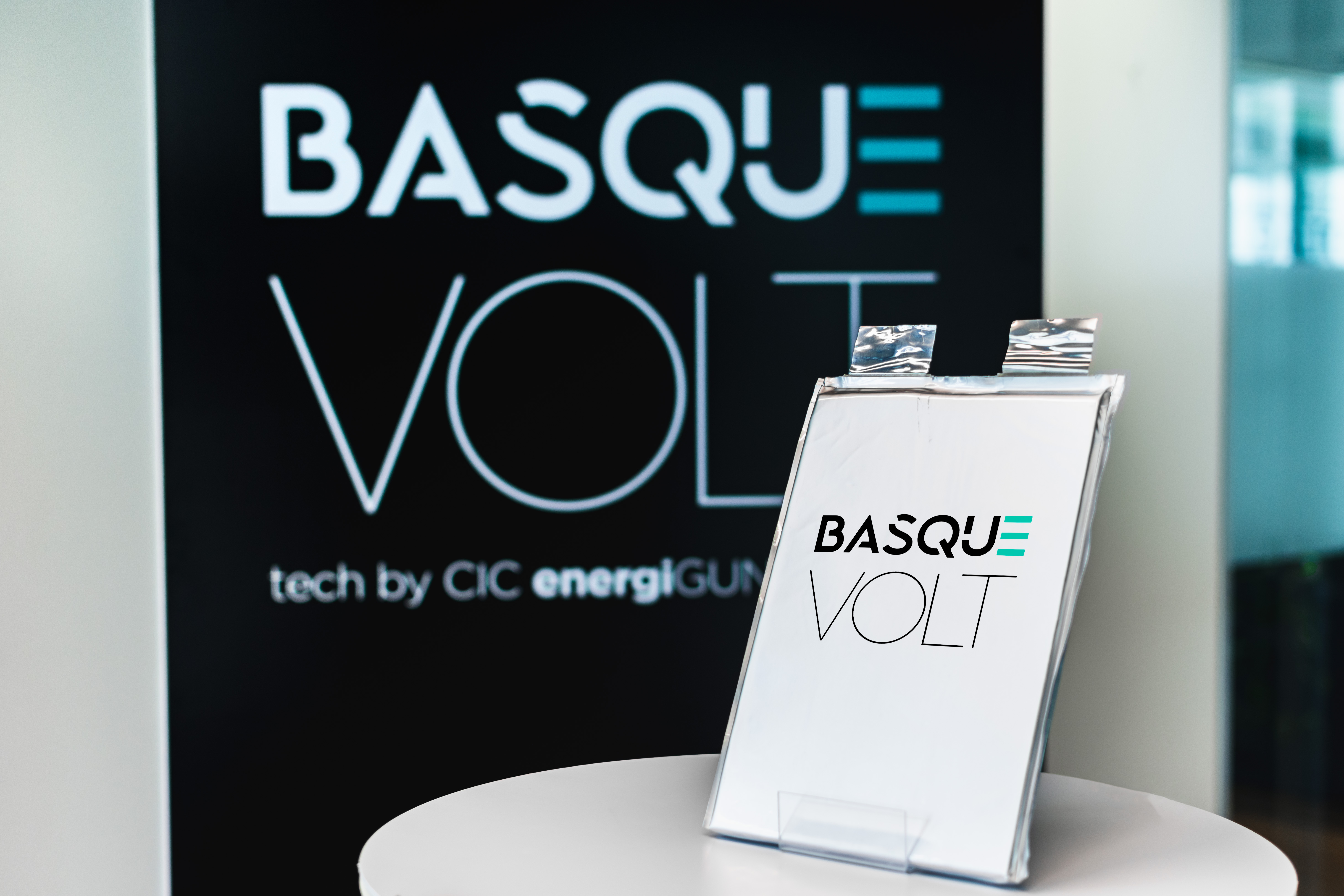
>> Check out the vendors in solid-state battery manufacturing on Qurator.com here
Sodium-Ion Batteries: Charging Ahead With Salt Power
Sodium-ion batteries offer an intriguing alternative, leveraging the abundant and inexpensive nature of sodium compared with lithium. This technology operates on similar principles to lithium-ion batteries, where sodium ions shuttle between the cathode and anode during the charging and discharging cycles. The appeal of sodium-ion batteries lies in their potential for low-cost and large-scale energy storage solutions, especially in regions where lithium resources are scarce or expensive.
While sodium-ion batteries typically suffer from lower energy densities than their lithium-ion counterparts, recent advances have led to promising improvements in performance, especially for stationary storage applications where size and weight are less crucial. Indeed, Swedish companies Altris and Northvolt recently succeeded in developing a sodium-ion battery cell with a best-in-class energy density exceeding 160 Wh/kg.
The ability of these batteries to operate over a wide range of temperatures and their stability over thousands of cycles make them suitable for grid storage and backup power applications. Furthermore, the use of more sustainable and less hazardous materials aligns with growing environmental concerns and the push for greener energy technologies.
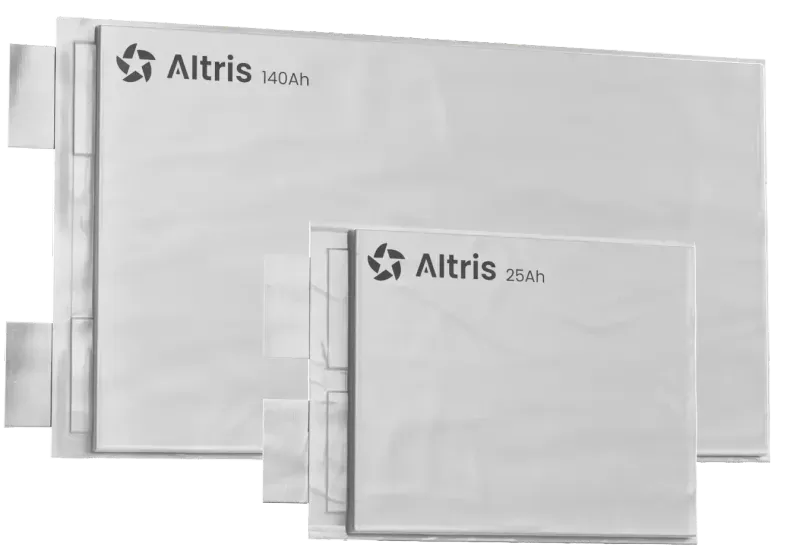
>> Check out the vendors in sodium-ion battery manufacturing on Qurator.com here
5. Conclusion: Batteries and the Road to Decarbonization
Battery chemistries have greatly evolved in recent decades and they continue to evolve at a rapid pace. This progression is not merely a technical achievement but a vital component in our global shift toward greener energy solutions and climate resilience. The intricate science behind battery chemistries is pivotal for the development and deployment of renewable energy technologies, thereby significantly contributing to our journey toward a carbon-neutral future.
At the heart of this evolution are remarkable advances in the materials and processes used in batteries, which have led to more efficient, durable, and environmentally benign energy storage solutions. By understanding and harnessing these chemistries, we can pave the way for a future that not only meets our energy needs but does so in a way that is harmonious with the environment and conducive to achieving carbon neutrality.