1. Introduction
In the rapidly evolving world of renewable energy, the quest for efficient and reliable ways to store and release electricity has never been more critical. Solid-state batteries are a class of battery technologies that differ significantly from the more common lithium-ion batteries found in many of today's electronic devices and vehicles. Unlike other types of batteries, which use a liquid or gel-like electrolyte, solid-state batteries utilize a solid electrolyte as the medium carrying ions through the battery.
The working principle of a solid-state battery is similar to that of any conventional battery — it involves the flow of ions between the anode and cathode to provide electric current. However, the solid nature of the electrolyte offers several advantages, such as better safety and higher energy densities.
2. How Do Solid-State Batteries Work?
In a solid-state battery, the solid electrolyte is sandwiched between the anode and cathode, where it allows ions to pass through but not electrons. During charging, the application of a voltage across the battery causes lithium ions to move from the cathode to the anode through the solid electrolyte. During discharge, this process is reversed: the lithium ions flow back from the anode to the cathode, releasing their stored energy as electricity that powers connected devices.
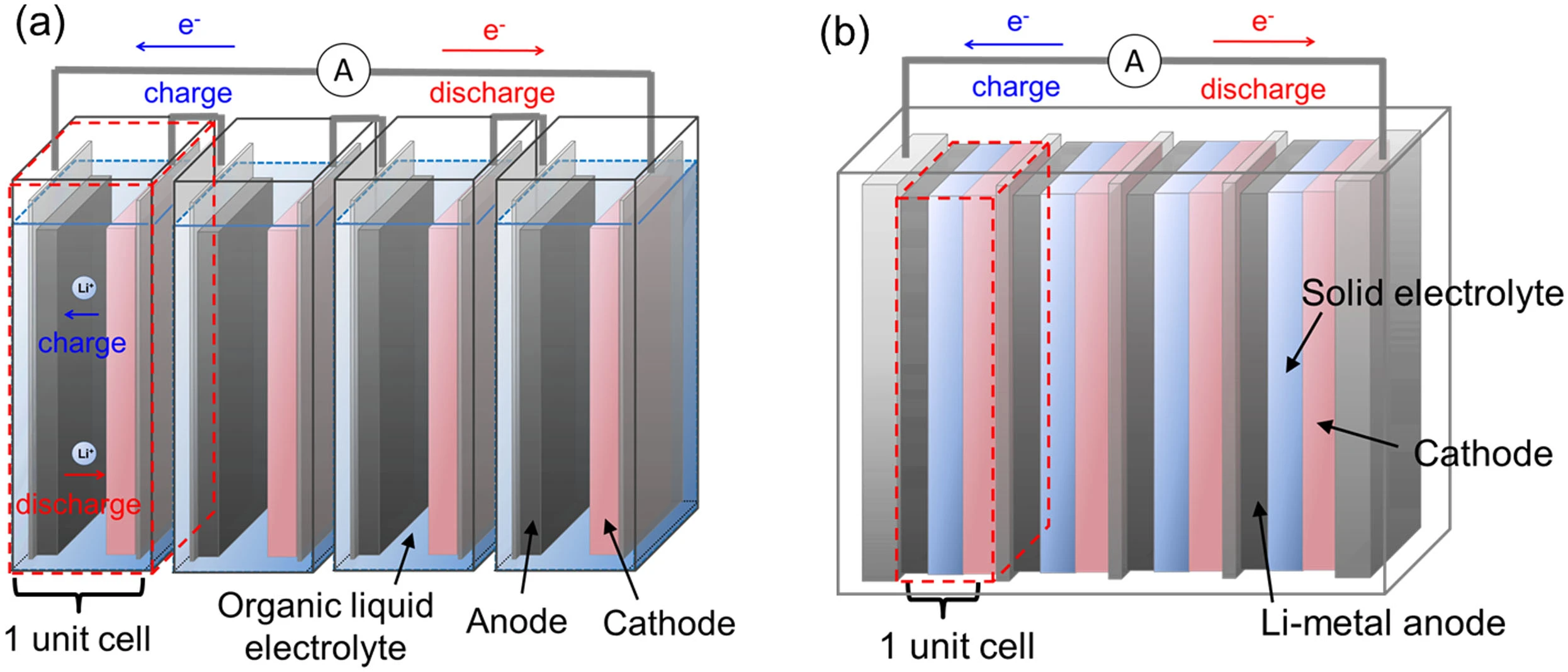
Comparison of a conventional lithium-ion battery (left) and a solid-state battery (right). Each battery is composed of four identical cells. Image source: Gambe et al., "Development of Bipolar All-solid-state Lithium Battery Based on Quasi-solid-state Electrolyte Containing Tetraglyme-LiTFSA Equimolar Complex", distributed under a CC BY 4.0 license
The cathode may be composed of lithium-based oxides, phosphates, or sulfides, while the anode is often made from lithium metal, which it is hoped will facilitate a substantially higher energy density compared with other battery technologies.
3. How Did Solid-State Batteries Come About?
Solid electrolytes themselves have a long history. In fact, they were first discovered as far back as the 1830s by Michael Faraday, but it is only recently that they have started to become viable candidates for widespread adoption in batteries. A major and longstanding bottleneck was that the solid electrolyte must exhibit high ionic conductivity to facilitate efficient ion transport while maintaining electronic insulation to prevent short circuits.
It was only in 2011 that Japanese researchers developed the first solid electrolyte with an ionic conductivity rivaling those of liquid electrolytes at room temperature. This material, lithium germanium phosphorus sulfide (LGPS), finally opened the door for solid-state batteries to compete with other battery chemistries. However, LGPS itself is chemically and electrochemically incompatible with lithium metal anodes and high-voltage lithium-ion cathode materials, severely limiting its applications in solid-state batteries.
Nonetheless, this breakthrough motivated a new search for solid electrolytes with the right balance of properties. Solid polymers, glass, and ceramics such as oxides, phosphates, and sulfides are among the key candidates. Common examples include lithium phosphorus oxynitride (LiPON), ceramics such as lithium lanthanum zirconium oxide (LLZO), and sulfide compounds that, despite their sensitivity to moisture, show promise owing to their high conductivity and flexibility. This is still an evolving field, and no consensus has yet been reached as to the optimal electrolyte.
4. Advantages and Disadvantages of Solid-State Batteries
The ultimate goal is that shifting to solid electrolytes will address several limitations of liquid and gel-like electrolytes.
Advantage #1: Chemical and Thermal Stability
- Solid electrolytes are inherently more stable, both chemically and thermally, extending battery lifespan and reducing the risks of leakage, evaporation, and fire.
- This superior stability could also enhance operational efficiency over a wider temperature range, as opposed to conventional batteries that can suffer from reduced performance in extreme cold as well as overheating at higher temperatures.
- The all-solid construction may also allow for more compact battery designs, because there is no need for bulky separators or the extensive safety features required to contain liquid electrolytes.
Advantage #2: Enables the Use of Lithium Metal Anodes Without Dendrite Formation
- Dendrite formation is a common issue in liquid electrolyte batteries where tiny lithium deposits can grow through the electrolyte, causing short circuits and potentially catastrophic failures.
- Using lithium metal anodes without worrying about dendrite formation is expected to permit a huge increase in energy density — perhaps to beyond 1,000 Wh/kg, or several times higher than that attainable with conventional lithium-ion batteries.
- This would in turn mean a smaller and lighter battery for the same output or a higher capacity for the same weight and volume.
- Overall, these advantages could result in safer, more powerful, and more versatile batteries.
Disadvantages: Transferring Theoretical Advantages to Practical Commercial Scale
Nonetheless, several problems have yet to be overcome. Although solid-state batteries are often touted as having the potential to reach higher energy densities than traditional lithium-ion batteries, this theoretical advantage still needs to be demonstrated in practical, commercial-scale batteries. The development of solid electrolyte materials with the desired characteristics — high ionic conductivity coupled with thermal, chemical, and electrochemical stability, mechanical strength, processibility, good compatibility with electrode materials, and low production cost — has so far proved extremely difficult.
- One particular set of challenges relates to the interfaces between the solid electrolyte and solid electrodes, where good contact is crucial for effective and reliable ion transport.
- As an emerging field, manufacturing processes must also be developed and optimized to decrease production costs and ensure economic viability.
5. Applications and Recent Developments in Solid-State Batteries
Medical Implants and Wearable Electronics
Solid-state batteries hold the promise of revolutionizing a wide range of industries owing to their higher energy densities, superior safety profiles, and longer lifespans compared with traditional batteries based on liquid electrolytes. It is now over 50 years since pacemakers based on solid-state lithium/iodine-polyvinylpyridine batteries were first implanted into patients, saving countless lives. Although these are primary batteries that are not rechargeable, there have been recent efforts to develop rechargeable alternatives for pacemakers and other medical implants, such as the Ilika Stereax line. These batteries are also finding their way into wearable electronics and Internet of Things devices.
First Forays into Electric Vehicles
More recently, solid-state batteries are being increasingly considered for electric vehicles, where their higher density and better safety could increase vehicle range and consumer acceptance. In fact, solid-state batteries have been used in some electric vehicles since as early as 2011, when the Bolloré Bluecar was launched in Paris, initially under a car-sharing scheme called Autolib' and later as a retail version. However, outside of a select few cities and car-sharing schemes, some of which subsequently closed down after failing to achieve scalability, the Bluecar has not become widely available. Despite being a pioneer, one limitation of the lithium metal polymer battery used in the Bluecar is its operating temperature range of 60 to 80 degrees Celsius, necessitating heating of the battery for adequate performance and highlighting the ongoing technical issues with such batteries.
Nonetheless, in 2015, Bolloré subsidiary Blue Solutions introduced the first commercial solid-state battery designed for use in electric buses and industrial vehicles, where continuous operation makes the temperature requirement less of a problem. This solid-state battery is now in its third generation, with the fourth-generation model set to be released in 2028.
Further Investments By the Automotive Industry
According to the Volta Foundation's 2023 Battery Report, most major automobile manufacturers are now actively investigating the use of solid-state batteries in their vehicles through a combination of in-house research, strategic partnerships, and investments, although the estimated start of production was described as "a moving target" in many cases. For example, Toyota announced last June that it had made a breakthrough in solid-state batteries and was aiming for commercialization in 2027–2028, although this was subsequently revised to "2030 and beyond" and approximately 10,000 vehicles just several months later in November.
In July, ProLogium and MAHLE signed a memorandum of understanding to develop a solid-state battery pack for electric vehicles based on a ceramic electrolyte. Toward the end of last year, Factorial Energy and Solid Power both delivered their first samples of solid-state battery cells to automotive manufacturers for testing, the former reportedly having a capacity exceeding 100 Ah. Just last month, U.S. company Ampcera announced that it had achieved a fast-charging milestone with its solid-state battery technology by demonstrating charging from 0% to 80% within only 15 minutes — crucial for improving the adoption of electric vehicles.
In October, Chinese automaker Nio revealed plans to include solid-state batteries in up to 14 of its electric vehicle models through its partnership with battery supplier WeLion, although WeLion's current offerings appear to use a semi-solid electrolyte rather than a true solid one. In January 2024, Nio also teamed up with other major automotive and battery companies in a government-led consortium aimed at establishing a supply chain for solid-state batteries by 2030 and securing China's dominant position on the global electric vehicle battery market.
Global Expansion and Mass Production
Initial efforts at mass production are also now underway. In May 2023, Ganfeng Lithium announced that it had begun mass production of its first-generation solid-state battery, which is based on an oxide ceramic electrolyte and reportedly exhibits an energy density of 260 Wh/kg. In January of this year, ProLogium opened the world's first gigafactory for solid-state batteries in Taiwan. The electric vehicle batteries being produced here are based on a high-silicon anode with a reported capacity of 106 Ah. The new facility will also assist in the design of ProLogium’s planned 5.2 billion euro gigafactory in France, signifying the start of major investments in solid-state battery production.
6. Conclusion
As the world moves toward decarbonization and a low-carbon economy, solid-state batteries represent a promising technology providing a more advanced means of energy storage and utilization. Their inherent advantages over current battery technologies — such as higher energy densities, improved safety profiles, and longer lifespans — are set to make them a useful ally in the transition from fossil fuels to renewable energy sources.
By improving the range, performance, and safety of electric vehicles, solid-state batteries could accelerate their adoption by drivers and encourage a move away from fossil-fuel-powered vehicles, a major source of global carbon emissions. This is crucial for reducing our reliance on carbon-intensive energy sources and replacing them with the renewable alternatives essential for mitigating climate change.
As these innovative batteries move from the research labs to the marketplace, their role in facilitating a seamless transition to a low-carbon economy is becoming increasingly evident. By enabling more efficient use of renewable energy and supporting the green transportation revolution, solid-state batteries stand at the forefront of our collective endeavor to mitigate climate change. This positions them as an important technology in the urgent and necessary shift toward a more sustainable and environmentally friendly future.